As many as 500 million people live in the semi-arid regions of the world, and most of them depend on agriculture for their livelihood. The populations of many of the countries in such regions have doubled in the past three decades and are still growing rapidly. These increases have not been accompanied by similar rises in food production. The pressing need to assure an adequate and reliable food supply is obvious. The dominant characteristic of the semi-arid zone is an insufficient water supply to support stable agriculture. Not only is there insufficient rainfall, but its occurrence is also highly erratic between years, during the year, and spatially, during any single rainfall event. In general, the rainfall pattern becomes more variable as the mean annual rainfall decreases. The semi-arid zone includes climates of two main kinds: the Mediterranean climate, where rain falls during the cool season of the year, and the semi-arid tropics where most or all of the rainfall occurs during the warm summer months. The minimum annual rainfall necessary to support a crop in the Mediterranean zone is approximately 250-400 mm, while in the semi-arid tropics it is 400-600 mm or even higher.
It is in the sub-humid to semi-arid tropics, however, that the problem of sealing, hardsetting, or soil capping, appears to be the most serious. Such conditions are typically found in the Sudan-Sahelian region of West Africa as well as in large parts of eastern and southern Africa, India, Thailand and elsewhere. Where the bare soil surface is sealed rainfall cannot penetrate and runs off laterally, even on very gentle slopes.
In many such localities the overall climatic conditions could allow one annual crop in most years. A 3-month rainfall of 700-1000 mm is sufficient for economic yields of crops like millet, sorghum, cowpeas and groundnuts. The success of dryland agriculture depends, however, on whether this rainwater can penetrate and be stored in the soil.
Moisture storage is usually not a restriction, unless the soils happen to be very sandy or very shallow. Many of the soils in the semi-arid tropics have effective depths of approximately 100 cm, with a subsoil structure and a clay minerals assemblage which guarantee an effective soil moisture storage capacity of at least 100 mm. A critical factor, however, is the degree to which the surface allows the rainwater to penetrate, right from the start of the rainy season. A significant part of the water does not enter the soil even though the water storage capacity of the soil is far from taken up. It is not generally realized that runoff losses from a field can amount to 30-35% of storm rainfall (Hoogmoed and Stroosnijder 1984). Much of the rain in semi-arid zones falls at high intensities, causing runoff and severe erosion. Since African rainstorms are usually heavy, such erosion is common even on moderate slopes. Sealing also impedes seedling emergence, because of the strength needed to break through the crust, and the formation of an oxygen-deficient layer immediately below the crust. When trees and bushes are cut, leaving the soil surface bare and unprotected, crust formations develop rapidly, preventing adequate moisture from entering the soil. This is the main cause of the progressive desertification in the Sudan-Sahelian region.
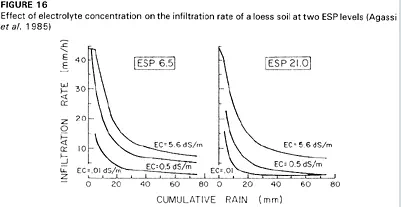
TERMINOLOGY
There are three main kinds of crusts and seals. These are described below.
Surface seal is defined as the orientation and packing of dispersed soil particles which have disintegrated from the soil aggregates due to the impact of rain drops. By definition surface seals are formed at the very surface of the soil, rendering it relatively impermeable to water.
A structural crust is defined as a surface layer of the soil, ranging in thickness from a few millimetres to a few centimetres, which is much more compact than the material beneath. The import of external materials is not involved in the formation of the crust. Structural crusts are formed also by physical forces as a result of trampling by livestock or through traffic by agricultural machinery and other vehicles.
Depositional crusts form when soil particles, suspended in water, are deposited on the soil surface as the water infiltrates or evaporates. Externally-derived materials are always involved in the construction of depositional crusts.
FACTORS AND MECHANISM OF CRUST FORMATION
The processes and mechanisms of crust formation are discussed in this section. Special emphasis is given to surface seal, which is dominant in most cultivated and exposed soils.
Surface Seal
The rapid drop in infiltration rate of most bare soils during rainstorms is due mainly to the formation of surface seal. The permeability of the seal is lower by several orders of magnitude, than the subsurface permeability. Surface sealing, as well as most other crust formations, results from three processes (Agassi et al. 1981; Morin et al. 1981).
- Physical disintegration of soil aggregates and their compaction, caused by the impact of raindrops.
- Chemical dispersion of the clay particles. The low electrical conductivity of the rainwater as well as the organochemical bonds between the primary particles of the surface aggregates, dictate the rate and degree of dispersion.
- An interface suction force which arranges suspended clay particles into a continuous dense layer. Such almost impermeable layers form right on the surface of the soil or in the immediate subsurface washed-in layer, as discussed by McIntyre (1958).
The separation above is artificial. The marked reduction in infiltration rate depends on the combined action of the three processes.
Figure 16 shows the relative influence of raindrop impact and chemical processes (Agassi et al. 1985). It stresses the importance of the electrical conductivity of rainwater and the high levels of soil sodicity in reducing infiltration rates. Since high electrolyte rainwater has an impact force only, eliminating the dispersion effect of the distilled water, the relative influence of these two processes can be seen. In general the beating action of the drops enhances the chemical dispersion of the soil.
FIGURE 16
Effect of electrolyte concentration on the infiltration rate of a loess soil at two ESP levels (Agassi et al. 1985)
Rain characteristics and their effects on surface seal formation
Rain impact forces depend on size distribution of the raindrops, their velocities and intensities. Table 11 gives such information for major rain types. It shows that light rain has a very low impact force compared with heavy rain. The low impact results from the smaller drop size (and hence velocities) and the lower rain intensity.
The relationship between rain characteristics and infiltration rates can be studied using a rainfall simulator. The relationships between soil infiltration, rain depth and rain momentum are demonstrated by Figures 17 and 18. Figure 17 presents the decline in infiltration as a function of cumulative rain depth, generated by different drop sizes and velocities, while Figure 18 presents it as a function of accumulated momentum. Both figures demonstrate that the decline of infiltration rate is greater and the final values are lower, with increase in height of the drops’ fall. Figure 19 presents the final infiltration rate as a function of momentum per kg of mass (1 mm m-2). The final infiltration rate of the loess soil decreases asymptotically as the impact of the drops increases. There are very small differences for the momentum values higher than 5 N s-1.
TABLE 11
Kinetic energy and number of drops for rainfall of various intensities (from Lull 1959)
Type of rainfall | Intensity (cm/h) | Median diameter (mm) | Fall velocity (m/sec) | Number of drops (n/m2/sec) | Kinetic energy/area unit time (joules/m2h) |
FogMistDrizzleLight rainMod. rainHeavy rainExces. rainCloudburstCloudburstCloudburst | 0.0130.0050.0250.0100.381.54.110.010.010.0 | 0.010.100.961.241.602.052.402.854.006.00 | 0.0030.0214.14.85.76.77.37.98.99.3 | 67 425 69627 0181502804954958201 215440130 | 5.9 x 10-71.2 x 10-32.212623.4 x 1023.2 x 1033.3 x 1034.0 x 1034.4 x 103 |
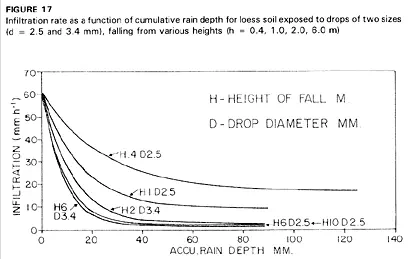
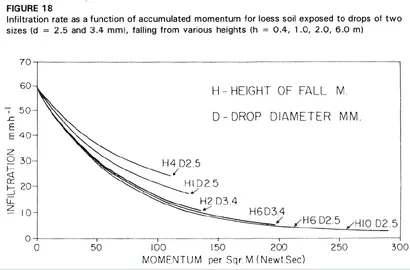
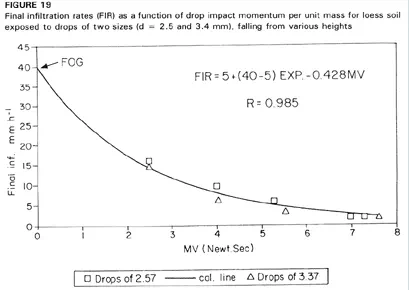
The infiltration and raindrop momentum relationships can be summarized as follows: the impact momentum of raindrops is a key factor in the quantitative evaluation of soil infiltration. When considering seal formation, a differentiation should be made between the rate of seal formation and the final permeability of the seal. The rate of seal formation depends on the accumulated momentum of raindrops beating the soil surface. The soil surface consists of aggregates of various strengths and drops with low impact are not able to break the more stable aggregates so they are not as effective in forming continuous impermeable seals. On the other hand, there is a threshold of the impact momentum values, and no further destruction of the aggregate is achieved by applying higher momentum force. Therefore, surface seal relations can be evaluated quantitatively using the rainfall momentum characteristics. The marked impermeability of surface seals in most African soils is directly related to the high impact values of the rainstorms there.
Structural Crusts
Hardsetting Crusts
Hardsetting of cultivated soil is a process of compaction, with increase in bulk density, that occurs without the application of an external load. In practice, it is difficult to distinguish between the effects of an externally applied load and the internal effect caused by the wetting of weak unstable soil. In previously loosened topsoil, during and after wetting, hardsetting involves the collapse of some or all of the aggregated structure (tilth). The hardsetting processes can be divided into two physically distinct processes: slumping and uniaxial shrinkage.
Slumping: Slumping is not limited to hardsetting soils. It occurs during and after the wetting of a soil horizon formed of water-unstable aggregates. The aggregates soften and swell simultaneously, and some or all of the silt and clay-sized material becomes suspended (although not necessarily as individual particles). Under appropriate ionic conditions, some of the clay fractions disperse. Aggregates disintegrate because they have insufficient strength to withstand the stresses set up by rapid water uptake, caused by rapid release of heat on wetting, trapped air, the mechanical action of rapidly moving water (Collis-George and Greene 1979), or by differential swelling (Emerson 1983). The matric potential of the soil, prior to wetting, also influences the incidence or severity of slaking. Moist aggregates slake less readily than air-dry ones because they have already completed some or all of their swelling and some pores are already water-filled.
Uniaxial shrinkage: Shrinkage is of importance because the closer proximity of particles that it entails makes a contribution to the increase in strength upon drying hardsetting soils. Laboratory experiments on the behaviour of aggregate beds of a hardsetting soil, wetted under tension or at zero potential, show that, at least during the early stages of drying, uniaxial shrinkage occurs. Since uniaxial shrinkage is, by definition, anisotropic, it follows that it must be accomplished by realignment of the disrupted aggregates and/or the internal fabric of the soil. Such a realignment can occur without cracking only if the forces holding the soil together are long-range and nonspecific. this point is important because this kind of force is likely to be provided by the matric potential, and therefore, when uniaxial shrinkage occurs, this may be an indication that effective stress contributes a dominant component of soil strength.
Mullins et al. (1987) have proposed the following explanation for the development and increase in strength observed in hardsetting soils, starting with a cultivated bed consisting of dry aggregates:
- Wetting of the system mobilizes some or all of the silt and clay. This may occur through slaking and/or dispersion.
- During the early stages of drying, the mobilized material is carried behind the retreating water meniscus to occupy concavities on the surface of sand grains and any remaining aggregates, forming annular bridges between them. This phenomenon has been observed under the microscope by Kemper et al. (1987).
- As drying proceeds, despite air entry into the soil, the mobilized material remains saturated until a very low potential is reached; consequently, the contribution of matric potential to the effective stress provides a major component of the soil strength.
Traffic Crust
Traffic crusts are discussed only briefly as they are outside the general scope of this article. Surface compaction by farm machinery and animals can cause a serious reduction in penetrating water and seedling emergence. Overgrazing can induce crust formation by two mechanisms:
- surface compaction of wet or moist soils; and
- mechanical destruction of the surface aggregates, which on dry soil can powder the soil surface.
This tends to speed up crust and surface seal formation at the oncoming of the rains.
Depositional Crusts
Depositional crusts, formed as suspended particles are deposited on the soil surface, are common in a limited area of cultivated and non-cultivated soils. The main sources of fine eroded soil particles are:
- Flood and furrow irrigation water
- Raindrop impact splash of loose soil particles from ridges and surrounding higher ground
- Overflow and floods from rivers
- Runoff and sheet erosion
The permeability of a depositional crust to water depends mainly on the clay mineralogy and the electrolyte concentration of the carrying water. The clay and silt particles in turbid suspension can either disperse or flocculate. They flocculate when the electrolyte concentration in the suspension exceeds the flocculation threshold of the clays (Oster et al. 1980). Depositional crusts formed from flocculated particles have an open structure and high permeability. Conversely, when the suspension electrolyte concentration is below the flocculation threshold, dispersed particles settle to form the depositional crust, the hydraulic conductivity of which is several orders of magnitude lower than that of the parent soil.
Comments are closed.